The North American grasslands stretch across the vast interior of the continent, occupying nearly 1.4 million square miles. We in the Midwest are proud of our section of the biome – the tallgrass prairie, which spreads from Manitoba south through the center of the continent to the Texas Gulf Coast, and reaches from Iowa eastward to Ohio and southern Ontario (Fig. 1). Like all biomes across the world, and other grasslands, the prairie was a product of climate, soils and the organisms that thrived in its environment. Most grasslands share several underlying environmental factors. Foremost is a climate that delivers 10-40 inches of annual precipitation, and which varies seasonally in a way that supports high primary productivity on one hand, and its subsequent senescence and accumulation as litter on the other. Here also, summer heat and thunderstorms combined with low humidity and warm winds to promote lightning-caused fires, an essential factor in making the tallgrass prairie. Ice storms and tornadoes helped thin tree canopies, and the presence of such browsers as buffalo, elk, and deer suppressed woody shrubs along grassland-woodland edges.
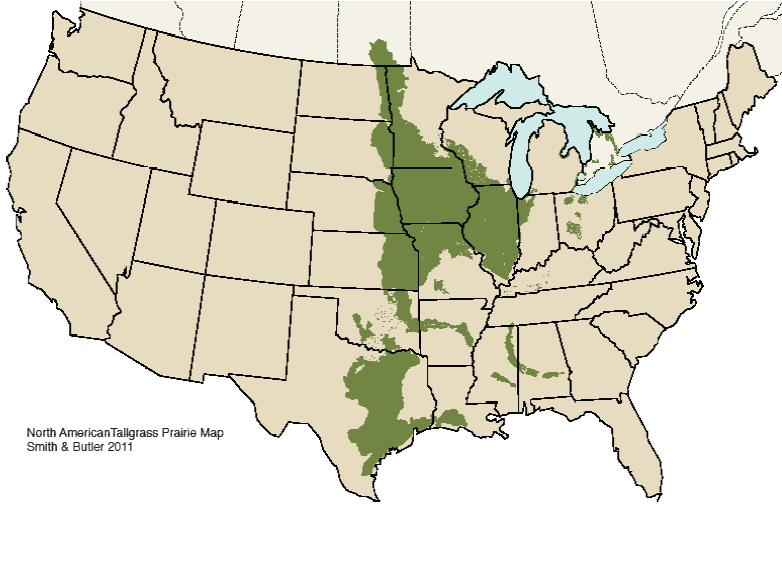
Figure 1. The tallgrass prairie ecosystem in North America.
Climate is the central factor distinguishing the Earth’s biomes from one another. (Fig. 2). Annual mean temperature and precipitation, along with their interaction, are key to understanding biome creation. So how has climate change—so often in the news nowadays—affected the making of the tallgrass prairie, over time?
First, it’s important to understand that climate change is far from a new phenomenon. In fact, it has been an enduring feature of Earth’s long 4.6-billion-year history. My goal with this paper is to use global climate change as a lens to explore its role in shaping what we now recognize as tallgrass prairie. I begin by asking two questions: 1) How have Midwestern environments, especially climate, changed over time? 2) How has plant migration, in response to climate fluctuation, shaped our perception of tallgrass prairie?
Before getting too far into these questions, though, there are a few important concepts we ought to review:
- Ecology is defined as the investigation (in biology) of the distribution and abundance of organisms in time and space. The more time or space we examine, the greater the likelihood there will be significant changes in the environment during that time or over that space.
- The most important influences acting on our extant prairies are those that are among the most recent. The further back in time one looks, the less impact there is on the present.
- Science’s ability to explain climate change diminishes the further back in time one looks. For the timeframes relevant to this article, we gather evidence of temperature fluctuation using various indirect methods, looking for patterns in temperature change evidenced in chemical and structural signatures in rocks, fossils, crystals, ocean sediments, fossilized reefs, tree rings, and ice cores (Scott and Lindsey 2014).
- The immense periods covered in this paper are foreign to our thinking and experience. We cannot begin to appreciate what a million years means in terms of day-to-day existence and the potential for environmental change and evolution, let alone what ten million or one billion years means.
Birth of earth to 1.5 billion years YBP
It’s safe to say that the climate of Earth’s first 3.1 billion years (67 percent of Earth’s history) had nothing to do with today’s tallgrass prairie. While Earth’s environment during this time is more mystery than certainty, there is evidence that temperatures were much warmer, perhaps 200 percent higher than now (some data suggest an ocean temperature in the Archean as high as 85°C, or 185°F) (Zalasiewicz and Williams 2012). This points to a very long-term trend in climate, with gradual cooling during the entire Precambrian. There are signs the cooling trend was punctuated by periods of deep cooling that caused glaciation, some which may have covered most of the Earth (Zalasiewicz and Williams 2012).
Other very important events are believed to have occurred during this time, some of them having had a direct effect on climate. First was the collision of Earth with a planetoid known as Theia, which not only produced our Moon and its tidal effects on Earth, but also affected the tilt and spin of the Earth. The collision is the cause of our planet’s seasonality and the day/night cycle.
Secondly, plate tectonics was born, causing continental drift and the subsequent formation of mountain ranges and ocean basins, both of which affect regional climates through rain shadows and ocean currents (Zalasiewicz and Williams 2012). Third, life on Earth began when prokaryotic bacterial cells evolved 3.5 billion YBP (years before present). The fourth crucial event was the oxygenation of the atmosphere by photosynthetic bacteria.
But a main point in this time period, highly relevant to the question of how climate change affected the evolution of the prairie, is that global climate change is represented by alternating periods of warm and cold, featuring glacial and interglacial phases. These phases occurred on Earth several times before Earth reached half of its current age and multicellular life evolved. We will see that these swings in temperature eventually became more prevalent in Earth’s history and very important to the tallgrass prairie.
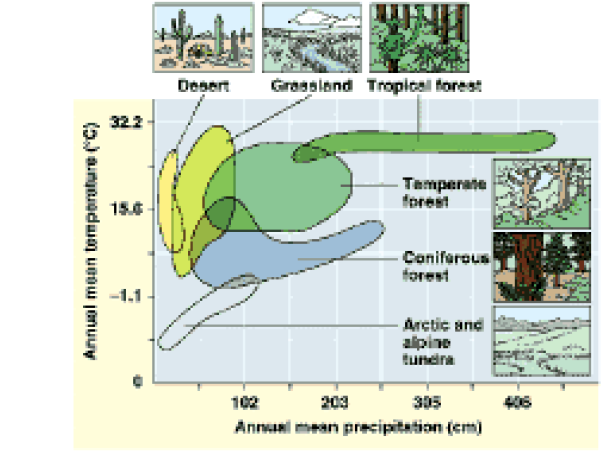
Figure 2. Climograph of North American biomes.
1.5 billion to 2 million years before present
This period of Earth’s history represents 99.87 percent of the last third of Earth’s 4.6-billion-year history. The starting point of 1.5 billion YBP is an arbitrary point, chosen because it represents the appearance of multicellular life forms. The end point of 2 million YBP marks the beginning of the Pleistocene epoch, the most recent period of recurrent ice ages. During this huge span of time, there were multiple events which had some bearing on Midwestern prairie. There is strong evidence the Precambrian ended with a series of glaciations—at 740 million YBP, 650 million YBP and 580 million YBP—and that the first two of these were especially cold with massive ice sheets (Zalasiewicz and Williams 2012).
The fluctuation between periods of glaciation—which lasted between 25 to 50 million years—and very warm ice-free periods, is characterized as fluctuation between icehouse and greenhouse. During an icehouse, continental glaciers grow and ebb in coverage during glacial and interglacial periods lasting in the range of one million years. In contrast, there are no continental glaciers present on Earth during a greenhouse. Three major icehouses have occurred during the last 540 million years—at 440 million YBP, at 300 million YBP, and the one beginning in the early Cenozoic (Zalasiewicz and Williams 2012, Brink 2015), which is the one we are still in.
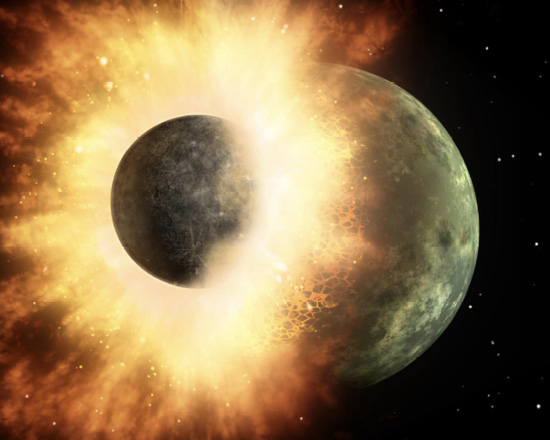
Theia colliding with the primordial Earth. From this collision came the moon and its tidal effects, as well as the Earth’s seasons and day/night cycle. Image from sci-news.com.
Considering the extreme levels of natural climate variation the Earth has repeatedly experienced, it becomes clear that global climate change has had a profound influence on earth’s history and all if its biomes. The environmental changes—from tropical during greenhouse to the absence of life on ice-covered land during an icehouse—caused massive movement, mixing and exposure to natural selection for much of Earth’s life.
The time periods during which these changes occurred is enormously important; the natural time frame for these changes is over tens of millions of years. The time frame for the climate change the Earth is experiencing now—driven by human activity—is on the order of decades.
Global climate variation, combined with continental drift over the first 500 million years of the Phanerozoic, was integral to the shifting pattern of marine, coastal and terrestrial environments that created the bedrock record of the Midwest. The exposure of bedrock is central to the ecology of limestone and sandstone prairies. It restricts soil depth, impacts soil fertility and increases surface runoff. Continental drift also played an important role in these changes, relocating the Midwest from the equatorial topics to its current temperate location with a continental climate. The tropical-like vegetation of the Late Carboniferous Period (318-299 million years ago)—the source of Iowa’s coal deposits—was forever lost, its presence now confirmed by fossils of Lepidodendron and Calamites (large tree-like plants that are now extinct).
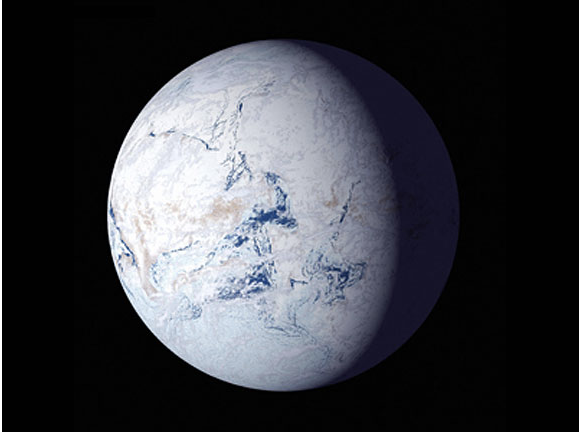
Indications are that, around 700 Million years ago, a “snowball Earth” covered in glaciers preceded the Cambrian Explosion of life. Image credit: NASA.
An event that was crucial to the making of the prairie and its climate occurred in the early Tertiary at the beginning of the Cenozoic. The Laramide Orogeny (Laramide in reference to the Laramie Mountains in southeastern Wyoming, Orogeny meaning folding of the earth’s crust to form mountains) was responsible for the rise of the Rocky Mountains between 65 and 40 million YBP. A rain shadow developed to the east of the mountains which gave the Great Plains the aridity that was essential to Midwestern grassland formation.
An extremely warm global event occurred during the Laramide Orogeny that reinforced the greenhouse that had been present through much of the Mesozoic. This spike in global temperature, lasting about 150,000 years, is called the Paleocene-Eocene Thermal Maximum (PETM) (Zalasiewicz and Williams 2012, Scott and Lindsey 2014, Hansen and Sato 2012). During the Mesozoic greenhouse, global surface temperature is estimated to have been around 25°C (77°F) on average. During the PETM, global surface temperature may have risen to 28°C (82.5°F). Considering that the current global surface temperature is approximately 14.5°C (58°F), the temperatures during the Mesozoic and early Cenozoic represent an Earth that was 24°F warmer than what we experience now. Tropical to subtropical environments, with palm trees and crocodiles, existed above the Arctic Circle (Scott and Lindsey 2014), with 74°F summer water temperature at the North Pole. Such temperatures, combined with a rain shadow that limited precipitation in the Great Plains and Midwest, surely increased aridity in the region, and fostered an environment very conducive to grassland formation.
Ten million years or so after the PETM, the Earth’s climate began a long-term cooling trend that initiated the current Cenozoic icehouse. Global surface temperature stabilized around 18° to 20°C (64° to 68°F, or about 9°F warmer than today) for roughly 35 million years, from the Oligocene through the Miocene to the early Pliocene, and the Antarctic ice sheet began to grow around 35 million YBP. Ashfall State Historical Park, near Orchard, Nebraska, provides a remarkable glimpse of this period, from the middle Miocene 12 million YBP. Fossil skeletons representing 13 mammal species, 2 bird species and 2 reptile species have been discovered, preserved in a deposit of volcanic ash that settled on this grassland/savanna ecosystem from an eruption in southwest Idaho.
Animal fossils, such as those at Ashfall, provide direct evidence of the fauna from past times, and indirect evidence of the flora and ecosystem present. The horses, camels, rhino, tortoises, deer and cranes found at Ashfall are species that nowadays are associated with grassland or savanna. When we combine our assumptions about the period’s ecology with the fossil evidence, it furnishes a more complete story. We know that the Midwest, from the middle Miocene to late Pliocene, was warmer than at present, and that it supported a grassland ecosystem. Direct evidence of the plant life also confirms that this grassland was a precursor to our present-day tallgrass prairie. Phytoliths are microscopic silica bodies that precipitate in and around cells in many plants. When plant tissues decompose, the silica is deposited in the soil, forming a record of past vegetation. An analysis of phytoliths from Nebraska and Kansas indicates that habitats varied substantially in openness during the middle to late Miocene, but became more uniformly open, corresponding to relatively open grassland or savanna, during the late Miocene and early Pliocene. There was also an increase of phytoliths characteristic of C4 grasses between 8 and 5 million YBP, which indicates the proportion of C4 grasses reached up to 50-60 percent of the grasses (Strömberg and McInerney 2011). C4 species use a more efficient type of photosynthesis than C3 species do—a type of photosynthesis that is especially adaptive in warm, dry environments. The dominant grasses of the tallgrass prairie—big bluestem, Indian grass, switchgrass, little bluestem—are C4 species. The climate and mountain-building of the early Cenozoic set the stage for the development of the North American grassland biome and the evolution of plant and animal characteristics.
Two million to 100,000 years before present
The Pleistocene epoch, beginning about two million YBP, and after about 50 million years of cooling trend since the end of the PETM, marks the beginning of a period of more intense cooling and a series of glacial and interglacial stages (Fig. 4). There is an amazing regularity in the record, where at least 20 glacial-interglacial cycles occurred at regular intervals of about 40,000 years until about 800,000 years ago when the interval became 100,000 years. The early cycles exhibited a temperature difference between glacial and interglacial of about 4°C (7°F), while the later cycles exhibited a temperature difference of about 9°C (16°F) (History of Earth’s Climate).
There are two useful observations to make about the Pleistocene climate record. First, there is a strong correlation between global temperature and CO2 levels (Fig. 5). While CO2 levels don’t necessarily tell us how climate change impacts the tallgrass prairie, CO2 levels do impact the growth rates of plants, competition between C3 and C4 plants, and water conservation in plants. The challenge is in the nature of the correlation—are both responding similarly to another factor, or is one factor actually dependent on the other?
The second observation is extremely relevant to the question of global climate change impacts on tallgrass prairie. The climate record documents an environment that displays very little if any long-term stability (e.g., tens of thousands of years) during the one million years or so that predate the emergence of the tallgrass prairie in the current interglacial. There must be a substantial ecological effect to any region and ecosystem that experiences this level of long-term climate fluctuation. In the next section, we will look into what that effect might have been.
100,000 years to present
This time frame encapsulates the last 88,000 years of the Pleistocene, which ended 11,800 years ago, and the Holocene, the epoch we are currently living in. Obviously, this time frame is very important, since it brackets the most recent portion of Earth’s history and furnishes the most accessible evidence for patterns of vegetation change over millennia. This evidence is most commonly and accurately provided by the science of palynology—the study of plant pollen, spores, dinoflagellates, and other microscopic “palynomorphs.” Palynomorphs are microscopic (5 to 500 µm) plant and animal structures composed of highly resistant compounds such as sporopollenin, dinosporin, or other related compounds (AASP—The PalynologicalSociety).
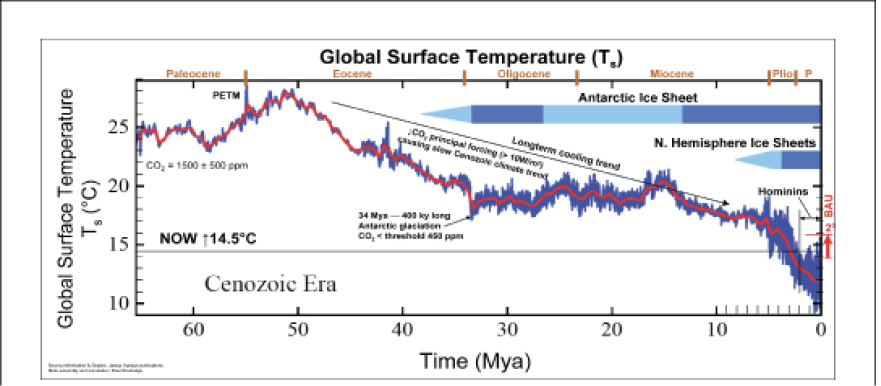
Figure 4. Areas of impacts due to Pleistocene glaciation in the United States. (From Dutch 1999)
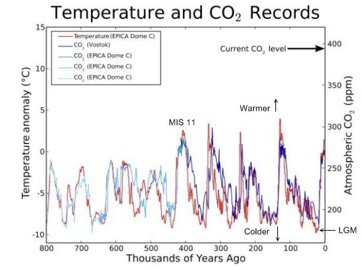
Figure 3. Global surface temperatures derived from Hansen and Sato (2012). Graph annotated and assembled by R. Routledge, PhD, President, Alpine Analytics (Deep Time: The Story of Mother Earth).
Palynology studies from all over the world furnish a collective body of data that has given us great insight concerning paleoclimates and ecosystems. Figure 6 provides a pollen diagram for a site in Illinois, on the eastern edge of the tallgrass prairie, which covers 14,000 years of vegetation (King 1981). Spruce parkland (grass pollen indicates some openness in the structure) was dominant 14,000 years ago, and was replaced a 1,000 years later with an ash-elm community that was more closed. The ash-elm ecosystem persisted until about 9,000 years ago, when oak-dominated woodland or savanna become more prominent. The coverage of oak woodland/savanna increased as the spruce parkland faded away, and was well represented on the landscape through recent time (at 1,000 years ago, the end of the time frame). It appears to have contracted somewhat between 5,000 and 3,000 years ago, coinciding with a rise in prairie. By 1,000 years ago, the oak woodland/savanna was dominant again, but this time a rise in the presence of weedy species (such as goosefoot, a species that colonizes disturbed ground) suggests that human disturbance was a more frequent factor (King 1981).
Plant migration and succession
The patterns of vegetation in the central Great Plains, where plant communities associated with a given location changed over time, exemplifies the concepts of plant migration and plant succession. Ecological succession is an ecosystem process that is central to the study of plant communities. It is a change in vegetation type over time due to an extended and consistent change in environment. Plant migration is the expansion of a plant species occurrence in space, caused by the movement of seeds and resulting in a change in the species range. Migration, for any organism, spans generations, so it encompasses both time and space. The series of plant communities present in the central Great Plains and Midwest from 32,000 to 2,000 years ago (Fig. 10) is the result of plants responding to environmental change. The warming climate was a primary factor in the environmental change, but other factors were likely important too—for example changes in the soil and disturbance caused by glaciation and the eventual deposition of glacial drift when the ice melted. Palynological studies have clearly shown that plant migration, induced by climate change, is a principal factor in understanding the prairie’s native plant communities.
Perhaps the single most important global climate event that has impacted the North American grassland biome, other than the rain shadow cast by the Rocky Mountains, is a relatively recent period in the Holocene, during which global temperatures climbed—the Holocene Climatic Optimum (HCO) (Fig. 7). There are different viewpoints on this period’s exact beginning and ending dates, but generally, the timeframe is between 8,000 and 5,000 years ago. It is the period of highest global temperatures since the last interglacial over 100,000 years ago. Figure 5. Global temperature and CO2 during the Pleistocene. Reconstructed from various sources, especially the EPICA Dome C Antarctic ice core. The cold spikes correspond to ice ages. The warm spikes correspond to interglacial periods like the present one (the Holocene). “LGM” stands for Last Glacial Maximum (From Peak Watch 2009).
The extreme warmth, abetted most likely by an increase in fire frequency, produced an environment more favorable to prairie than to forest. The “war” between grassland and forest (in terms of which ecosystem occupied the landscape), which played out along the edge between these two biomes, was won by grassland for 3,000 to 4,000 years. Plant migration of tallgrass prairie species pushed prairie eastward from what was then the heart of the prairie, into Indiana, Ohio and parts of Michigan and Pennsylvania, in what is referred to as the prairie peninsula (Fig. 8). At the same time the tallgrass prairie was expanding eastward, species from the mid-grass prairie in the Great Plains (the western and drier version of the central grassland biome) were also migrating in that direction, advancing into central Iowa. Pollen studies covering North America’s last 18,000 years suggest that the peninsula began 8,000 years ago. Eventually, as the Holocene climate cooled and the Midwestern environment become more cool and moist and the wild fires waned, the forest began to win the war with grassland. Forest encroachment reclaimed landscapes that had been lost during the HCO. Plant migration shifted the forest, tallgrass prairie, and mid-grass prairie back to the west, making a landscape more similar to the present.
Plant migration and the tallgrass prairie
A good case can be made that the global climate, with its proclivity to change over time, has at several times throughout Earth’s history played a role in shaping today’s tallgrass prairie. Fluctuating sea levels caused by fluctuating climate were integral to the formation of Midwestern bedrock layers (through formation of sedimentary rock in marine or coastal environments) and its effects on ecosystem soils and topography. Grassland expansion was favored by the climate which mountain-building brought about, and the climate during greenhouse conditions pushed plant evolution in the direction of C4 photosynthesis, an important characteristic of prairie grasses. Icehouse environments, with their pattern of alternating glaciation and interglacial periods, were responsible for much of the Midwest’s soil and topography. However, in the end, the most significant factors in this process of landscape creation are the most recent ones. Plants responded to climate change by migrating, and during the retreat and advance of glaciers over the last one million years, this has had dramatic effects on our tallgrass prairie.
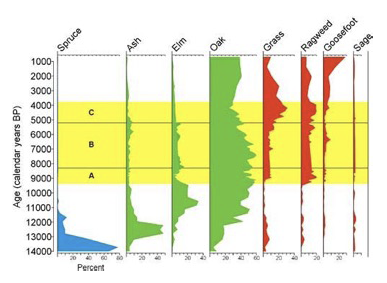
Figure 6. Pollen diagram from Chatsworth Bog, Livingston County Illinois (King 1981).
The central North American grassland biome—the precursor to tallgrass prairie as we know it—was in place for millions of years. Then a long sequence of glacial episodes occurred, during which both plant and animal species moved first southward to locations of refugia during glaciation and then most likely spread back northward during interglacial periods. The southward migrations would have occurred through secondary succession (succession on sites that after disturbance still retain soil, seeds, roots, and organisms), as colder weather removed sensitive species and created space for southward-moving species. Northward migrations would have incorporated primary succession (succession of sites disturbed so severely all biological legacy is removed), as melting glaciers exposed new geological deposits and landscapes.
Plant migration is fundamentally different from animal migration. It involves four processes: reproduction (seed production), dispersal of offspring, establishment (seed germination and seedling survival) and growth to reproductive maturity (to produce more offspring). Dispersal occurs via transport of seeds, spores or fruits. Anemochory (wind dispersal), endozoochory (inside of animals) and ectozoochory (on outside of animals) are the principal mechanisms plants use for dispersal. Because migration over long distances is a generational process, short-lived plants (annuals or biennials) can migrate faster than long-lived plants (perennials). The life history strategy of species, which describes its allocation of energy to survival, growth and reproduction, is central to how quickly a species migrates. Migration happens at the levels of individuals and of populations; communities do not migrate as units. Each species’ unique life history and niche affects its ability to establish and grow, and give it a unique migration behavior. As in a gigantic mixing bowl, where the ingredients are independently added and subtracted at different rates and from different parts of the kitchen, the mix of the region’s vegetation was produced by the complex interaction of species-specific migration behavior, different regional refugial locations (places species migrated to during ice advances to survive), and the long sequence of many glacial episodes in the north central U.S.
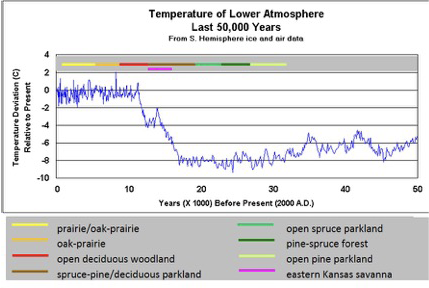
Figure 7. Global temperature data for the last 50,000 years from Satellite stratospheric data, Southern Hemisphere ground temperature data and Vostok ice core data (from Global Warming: A Chilling Perspective). Midwestern plant communities based on palynology studies (Fredlund and Jaumann 1987).
Implications for today’s prairie
The effect of this species mixing is twofold. One is that endemism (when a species is restricted to a single place, location or region) is very rare in the tallgrass prairie. Endemism is fostered by isolation, climate and environmental stability, as well as rapid rates of evolution and speciation. Madagascar, an isolated tropical island off the coast of Africa in the Indian Ocean, has one of the highest endemic rates—80 percent of its plant and animal species are endemic (found nowhere else on earth). Nearly all of the plant species that inhabit the tallgrass prairie also occur in other ecosystems in other parts of the country. There are three species that are exceptions, and their endemism in the tallgrass prairie is highlighted by their rarity. All three are federally threatened species—western prairie fringed orchid (Platanthera praeclara), slender prairie bush clover (Lespedeza leptostachya), and Mead’s milkweed (Asclepias meadii).
The second consequence of species mixing and migration is the establishment of geographically-restricted species—that is species which occur in only a specific part of a state or region (as with yucca in Iowa, which is restricted to the western tier of counties, but it is not endemic to Iowa because it occurs throughout the Great Plains). Most of the tallgrass prairie species in Iowa are geographically-restricted within Iowa; they are not distributed state-wide. This has very important implications in prairie reconstruction and the design of seed mixes. To ensure that reconstructed prairies function and look like remnant prairies, the species that are seeded should be native to that region of Iowa. A sense of place is imperative; simply being native to Iowa does not insure a sufficient level of accuracy in recreating a prairie. It’s the geographically-restricted species that furnish a prairie with its unique signature, a locally-determined factor that distinguishes it from other prairies in the state and expresses an area’s home-grown natural heritage. Since the biogeographical ranges of organisms do not recognize political boundaries, every state has geographically-restricted species.
Each species’ unique migration behavior means that species with adaptations that facilitate the greatest dispersal distance would be more likely to reach the Midwest and become a member of the tallgrass prairie. High dispersal may be one reason why the Aster family, which employs a feathery top to achieve wind dispersal (as the familiar dandelion does), is the most species-rich family represented in tallgrass prairie. Differential migration rates explain the biogeography of woody species in the Loess Hills along Iowa’s western border. Tree and shrub species that migrated northward from southern refugia after the last glaciation exhibit a range of success as one moves from south to north. There are 32 woody species present at the south end of the Loess Hills, but only 14 species at the north end. The difference has provided greater protection from woody encroachment in the north, where larger tracts of higher quality prairie are present.
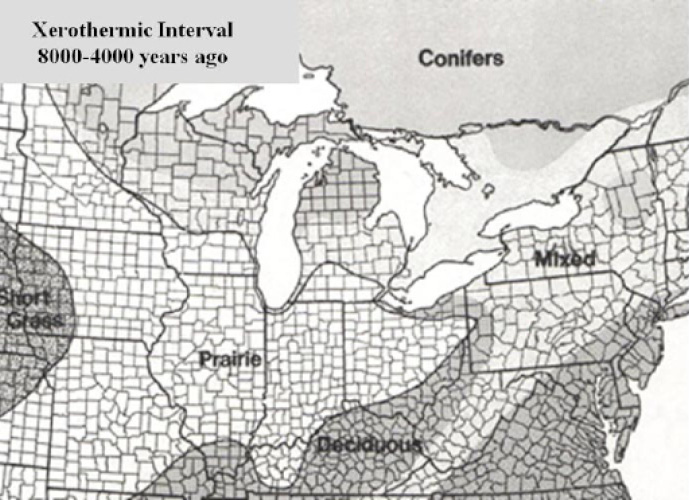
Figure 8. The Prairie Peninsula. (From Ohio Plants 2017).
A final consideration on the impact of plant migration on the prairie is the species migration associated with the prairie peninsula. The eastward shift of mid-grass prairie from the Great Plains into Iowa explains the occurrence of many western species in Iowa, especially in the Loess Hills. For example, dotted blazing star (Liatris punctata) and scarlet guara (Gaura coccinea) only occur in the Loess Hills and on dry microenvironments at a few other sites, also in the western third of state. These species probably arrived in Iowa during the Holocene Climatic Optimum (HCO) and spread throughout most of Iowa at that time. However, when cooler, damper environments returned, most populations died out except for those located in microenvironments (southwest slopes in the Loess Hills, dry gravelly soil on glacial moraines) that mimic the arid habitats in the Great Plains. Large-flower beard tongue (Penstemon grandiflorus), a species centered in the central Great Plains, also occurs in the Loess Hills and many other gravelly or sandy sites into eastern Iowa. It can also be found in disjunct populations on the sand dune ecosystems at the south end of Lake Michigan in Indiana. Its current biogeography, with outlier populations in Ohio and Michigan, seems to follow the prairie peninsula.
From all the evidence discussed, it is certain that global climate change and plant migrations have greatly impacted the Midwestern tallgrass prairie in its structure and composition, and possibly in other additional ways we can never know. Perhaps the most important outcome of the HCO and the expansion of the prairie peninsula is that prairie was firmly established in Iowa at a time (5,000 years ago) that is recent on a geological timescale. Mid-grass and tallgrass prairie likely covered all of Iowa by the end of the HCO, a period when it was very difficult to be a tree in Iowa. So even though climate in the Midwest has been favoring forest for the last 3,000 years or so, the prairie was so deeply entrenched in Iowa, its resilience and stability has enabled it to resist forest encroachment. This greatly helped to insure Iowa’s legacy as the heart of the tallgrass prairie, and it has furnished us with a rich array of prairie communities to appreciate, study, honor and protect.
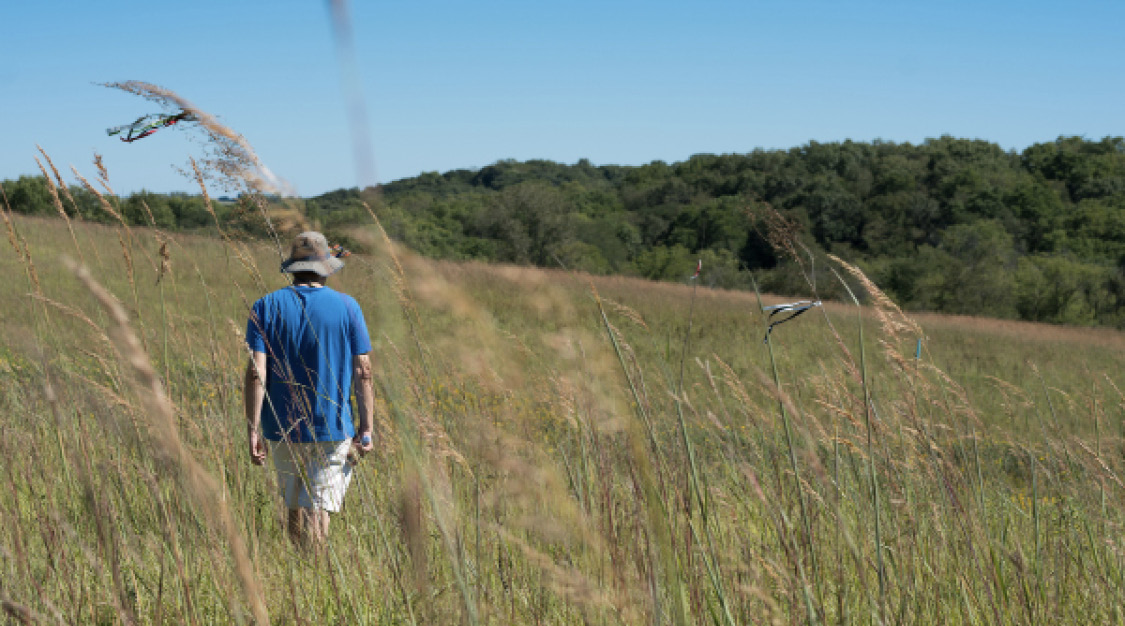
A visitor walks through the restored prairie at the College’s Conard Environmental Research Area (CERA). Photo by Jun Taek Lee.
Looking back into history and examining the patterns of global climate change on Earth has helped to address questions concerning function, process and pattern on our present-day ecosystems and landscapes. So what does the climate data suggest to us concerning the future? Out of the large body of scientific research on Earth’s past climates—some of which has been cited in this paper—two important philosophies emerge. First, natural climate change, with magnitudes much greater than humans have experienced, has been a consistent pattern in Earth’s history. During those changes, there must have been massive shuffling, mixing, crowding and killing of the Earth’s biodiversity during glacial advances. But each time, during periods of glacial retreat or greenhouses, life rebounded, and evolution likely produced species more adept at coping with the Earth’s fickle climate. Second, the time frame for these oscillations in climate—during which the amount of change was astounding—ranged from 50,000 to more than 10,000,000 years. Patterns in the Earth’s orbit around the sun and plate tectonics are the principal forces that drive long-term climate (Zachos et al. 2001). These are forces that proceed over incredibly long time scales; far beyond any individual’s concept of time. Current climate is changing at a much quicker rate. Global temperature, in just the last 100 years, has completely reversed a downward temperature trend that occurred over the last 7,000 years. It’s simply not possible for the climate change currently underway to be explained by the Earth’s natural cycles. We ought to be in a downward temperature trend—the pattern expected at the end of an interglacial. Instead, global temperature is increasing at rate faster than ever experienced. So the outlook for the future is somewhat disconcerting and ambiguous, except that temperature is likely to continue to increase and that time and evolution are probably not on our side. It is in its way, a grand experiment. What happens when vast amounts of carbon from Earth’s vast, long-term, carbon storage pools are released to the atmosphere? I usually like experimental study and exploring science to satisfy my curiosity. But this is one experiment most of us wish could be stopped.
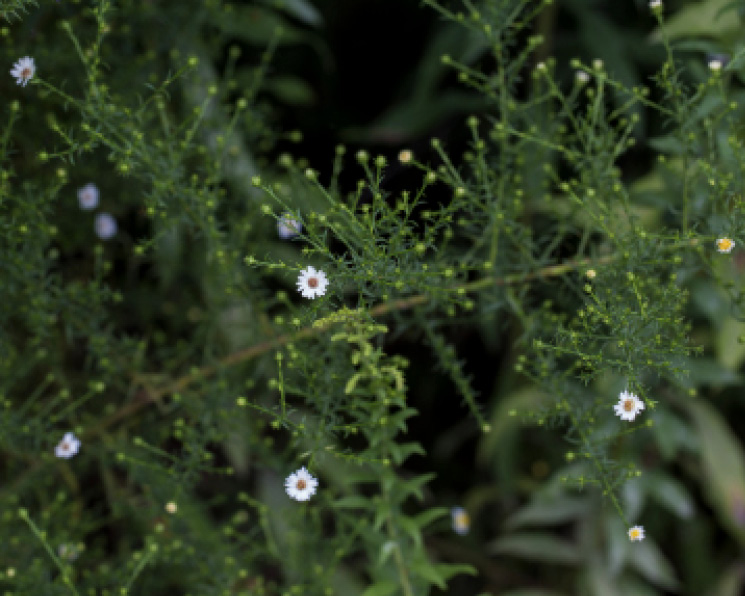
“the Aster family, which employs a feathery top to achieve wind dispersal (as the familiar dandelion does), is the most species-rich family represented in tallgrass prairie.” Photo by Justin Hayworth.